What ‘The Meg’ gets wrong — and right — about megalodon sharks
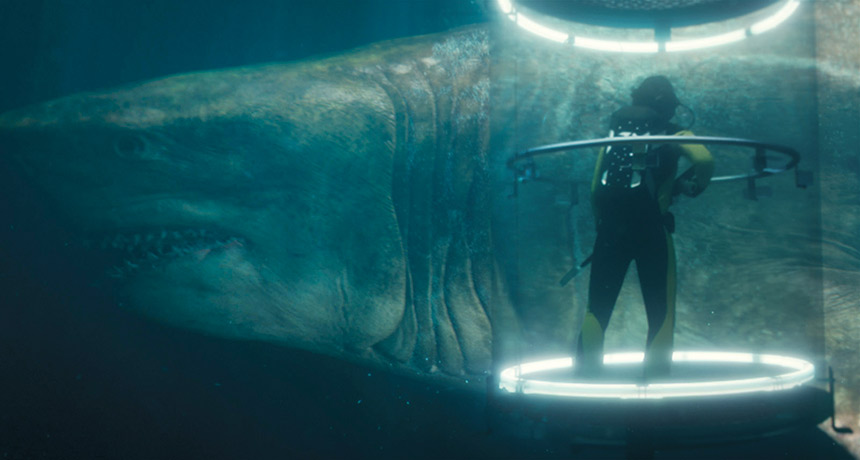
OK, so what if a giant prehistoric shark, thought to be extinct for about 2.5 million years, is actually still lurking in the depths of the ocean? That’s the premise of the new flick The Meg, which opens August 10 and pits massive Carcharocles megalodon against a grizzled and fearless deep-sea rescue diver, played by Jason Statham, and a handful of resourceful scientists.
The protagonists discover the sharks in a deep oceanic trench about 300 kilometers off the coast of China — a trench, the film suggests, that extends down more than 11,000 meters below the ocean surface. (That depth makes it even deeper than the Mariana Trench’s Challenger Deep, the actual deepest known point in the ocean). Hydrothermal vents down in the trench supposedly keep those dark waters warm enough to support an ecosystem teeming with life. And — spoiler alert! — of course, the scientists’ investigation inadvertently helps megalodons escape from the depths. The giant living fossils head to the surface, where they terrorize shark fishermen and beachgoers a la Jaws.
But could a population of megalodons actually have survived down there? To explore what is and isn’t possible and what we still don’t know about sharks, Science News went to the movies with paleobiologist Meghan Balk of the Smithsonian’s National Museum of Natural History in Washington, D.C., who studies the ancient predators.
Did megalodons ever actually get as big as they are in the movie? Extremely unlikely
The megalodon sharks of The Meg reach sizes of about 20 to 25 meters long, the film says — massive although just a tad smaller than the longest known blue whales. But estimates based on the size of fossil teeth suggest that even the largest known C. megalodon was much smaller, at up to 18 meters — “and that was the absolute largest,” Balk says. On average, C. megalodon tended to be around 10 meters long, she says, which still made them much bigger than the average great white shark, at around 5 to 6 meters long.
Would a megalodon otherwise look like the film version? Yes and no
The movie’s sharks aren’t entirely inaccurate representations, Balk says. These megalodons correctly have six gills — between five and seven is accurate for sharks in general, she says. And the shape of the dorsal fin is, appropriately, modeled after the great white shark, the closest modern relative to the ancient sharks. Also, a male meg in the film even has “claspers,” appendages under the abdomen used to hold a female during mating. “When I looked at it, I was like, oh, they did a pretty good job. They didn’t just create a random shark,” Balk says.
On the other hand, it’s actually a bit odd that the movie’s megalodons wouldn’t have evolved some significant anatomical differences from their prehistoric brethren, Balk says. “Like the eye getting bigger” to see better or becoming blind after a few million or so years living in the darkness of the deep sea, she says. Or you might even expect dwarfism, in which populations restricted by geographic isolation, such as being stuck within a trench, shrink in size.
Would such huge sharks have had enough to eat down there? Extremely unlikely
In general, “there’s just not enough energy in the deep sea” to sustain giant sharks, Balk says. Life does bloom at hydrothermal vents, although the deepest known hydrothermal vents are only about 5,000 meters deep. But even if there were vents in the deepest trenches, it’s not clear there would be enough big species living down there to sustain not just one, but a whole population of massive sharks. In the film, the vent field is populated with many smaller species known to cluster around hydrothermal vents, including shrimps, snails and tube worms. Viewers also see one giant squid, but there would have had to be a whole lot more food of that size. C. megalodon — like modern great whites — ate many different things, from orcas to squid. And the humongous megalodon sharks in the movie “would have eaten a lot of squid,” Balk says, laughing.
Could sharks live at such depths? Unlikely
How deep sharks can live in the ocean is actually still a big unknown (SN Online: 5/7/18). “Quantifying the depth that sharks go to is a big endeavor right now,” Balk says. Few sharks are known to inhabit the abyssal regions of the ocean below about 4,000 meters — let alone the depths of oceanic trenches lying below 6,000 meters. Aside from the scarcity of food, temperature is another limitation to deep-sea living.
Sharks that do inhabit deeper parts of the ocean, such as goblin sharks and the Greenland shark (SN 9/17/16, p. 13), tend to have low metabolic rates. That means they move much more slowly than the energetic predators of the movie, Balk says. C. megalodon, although it lived around the globe, tended to prefer warmer, shallower waters and used coastal regions as nursing grounds.
So, could megalodons have survived to modern times without humans knowing about it? Extremely unlikely
Sharks shed a lot of teeth throughout their lives, and those teeth are the main fossil evidence of the life and times of prehistoric sharks (SN Online: 8/2/18). Fossilized C. megalodon teeth found in sediments around the world suggest that the creatures lived between about 14 million and 2.6 million years ago — or perhaps up until 1.5 million years ago at the latest, Balk says. It’s not clear why they went extinct. But there are a handful of hypotheses: competition for food with other creatures like orcas; ocean circulation changes about 3 million years ago when the Isthmus of Panama formed (SN: 9/17/16, p. 12); nearshore nursery sites vanished; or possibly a loss of prey sources stemming from a marine mammal extinction about 2.6 million years ago.
Bottom line: The sheer abundance of shed teeth — as many as 20,000 per shark in its lifetime — is one of the strongest arguments against megalodon surviving into modern times, Balk says. “That’s one of the reasons why we know megalodon’s definitely extinct. We would have found a tooth.”